Chronic pain currently affects roughly 30% of the population in the United States. The most common method for managing chronic pain has traditionally been pharmacological. These treatments often include non-steroidal anti-inflammatory drugs and opioids. Currently, between 5 and 8 million people in the USA use opioids for chronic pain management. In light of the growing opioid crisis, The Centers for Disease Control and Prevention have emphasized the importance of using non-opioid analgesic drugs and techniques for acute and chronic pain management. Laser therapy is a non-invasive alternative to the pharmacological intervention that has demonstrated its effectiveness for both chronic and acute pain.
Lasers have been extensively used for therapeutic applications in Europe for more than 30 years. The FDA had cleared a low-level laser therapy (LLLT) in 2002, and the class IV therapy laser in the following year. While they are both appropriate for treating acute and chronic pain, the benefit of Class IV lasers is that they allow therapeutic doses of photons to be applied over larger areas to deeper tissues and in a shorter time, which is a significant consideration for most clinicians. The FDA approved indications for use of Class IV laser include the following:
- relief of muscle and joint aches, pain, and stiffness;
- relaxation of muscles and muscle spasms;
- temporary increase in local blood circulation; and
- relief of pain and stiffness associated with arthritis.
The primary effect, which is usually referred to as photobiostimulation, is hypothesized to stimulate the organism on the cellular level to enhance healing and recovery. The cytochrome c oxidase, a protein responsible for charge transport in mitochondria, is presumed to be the receptor site responsible for the beneficial effects of laser light because of its absorption peaks in the near-infrared wavelength range. Mitochondria are the power plants of the eukaryotic cell. It uses the energy released by the oxidation of glucose to produce ATP. Oxygen is processed in cells by mitochondria. Faster exchange of oxygen and metabolites due to laser irradiation causes more oxygen atoms to reach mitochondria. Mitochondria are further stimulated to synthesize ATP faster. The increased ATP in cells is then utilized as energy supply to carry out a variety of cellular activities and biochemical reactions that lead to faster recovery, healing and edema reduction in the treated area.
Another effect of High-Intensity Laser therapy is the absorption of the laser light by water and fat, which consequently cause a temperature increase in the tissue. Increase in tissue temperature causes vasodilatation. As a result, blood perfusion increases, larger amounts of oxygen are delivered to the tissue, accelerating metabolic processes and relaxing muscles. Pain caused by muscle tension is relieved, resulting in an immediate increase in range of motion.
There are indications that laser light decreases bradykinin, which is a physiologic mediator of pain. As bradykinin antagonists, the light has analgesic activity in both acute and chronic pain.
Despite extensive reporting of the successful application of lasers for treating various conditions, and strong evidence that laser light promotes tissue regeneration, reduces inflammation, and relieves pain, the mechanism behind clinical benefits of therapy lasers remains debatable. Moreover, there is no consensus regarding major physical properties of light, such as wavelength, power, power density and dose of irradiation that produce clinically beneficial results.
There is a lot of confusion in the medical world regarding laser properties that are important for treatment.
Wavelength
The laser wavelength is one of the most important specifications of the light source. Some wavelength may have a beneficial effect on the body, while others may not. Also, the penetration depth of light is strongly dependent on the wavelength. While shorter wavelengths (UV and blue) are absorbed within a fraction of a millimeter under the skin, longer wavelengths, such as red and infra-red may travel many times deeper, affecting tissue well below the surface.
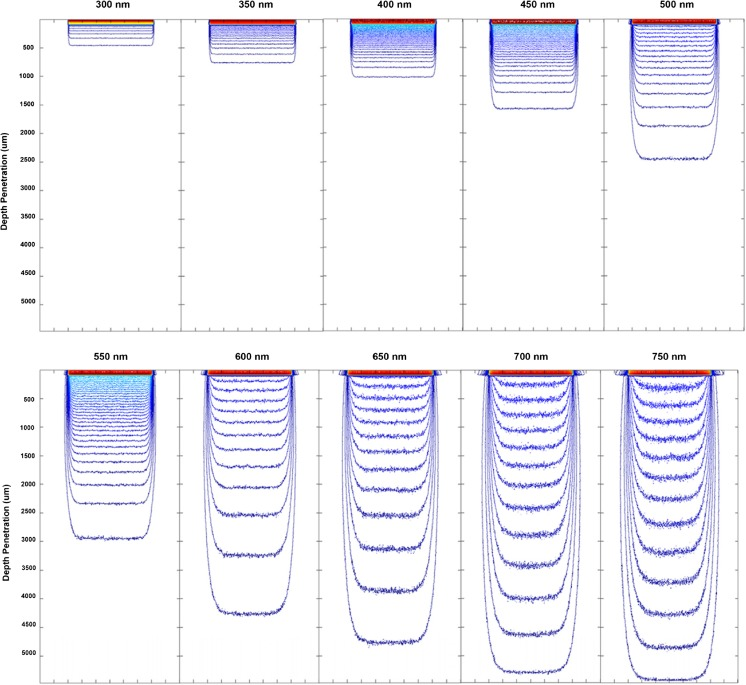
Power
Perhaps the most misunderstood specification is laser power. The laser power itself is irrelevant to clinical outcome. For example, a tightly focused beam of a 980nm laser diode with output power 1W will cut through the soft tissue, while the same laser shining a light on the 2-inch diameter spot on the skin will produce no sensation.
Power density
It is the power density that is more important. If the power density is low, the procedure may require long exposure in order to achieve a therapeutic effect, if any. On the other hand, excessive power density would cause discomfort or even pain. For a given wavelength and laser beam size, the higher power density allows light to penetrate deeper.
Spot size
While high power density can be achieved by reducing the size of the beam, this is not a good idea. Not only it will increase the duration of the procedure, as scanning over large treatment area will be required, but it will also introduce uncertainty in the dose of light. Spot size has important clinical implications due to its effect on penetration and light dispersion in tissue. With increasing spot size, there is a reduction in the amount of lateral scattering; this results in greater penetration for larger spot sizes. As a result, lower power densities can be applied when using larger spot sizes to achieve the same penetration depth for treatment (see Figure 2). It has also been found that although the penetration depth increases, there is a point at which the beam width has no further effect on the penetration depth and this is expected to be in the region of 5–12 mm in beam diameter. It is obvious from the picture that for a beam diameter less than 20mm the exposure becomes quite non-uniform, making control of the dose next to impossible.
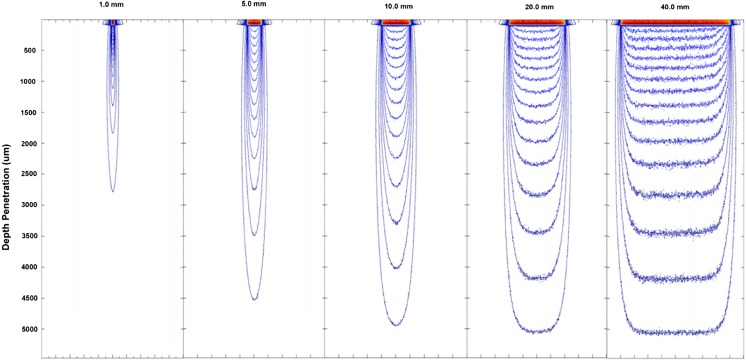
Treatment Dose or energy per unit area of tissue surface is a moot point in laser therapy. An astoundingly wide range from as low as 0.001 J/cm2 to as high as 24 J/cm2 has been reported. However, it is likely that many of the reported doses were calculated incorrectly. Not only because the laser equipment did not provide uniform illumination of the treated tissue, as described previously, but also because the calculation of the dose is quite complicated and depends on the type of tissue and its condition, pigmentation, treatment technique, etc. Moreover, there are vast differences in irradiating tissue cultures in a laboratory and treating deep-lying tissue in-vivo.
Akela Laser Corporation has developed most advanced laser diode sources for clinical research and practice. They are available at any wavelength, and with high power. Sophisticated delivery optics produces highly uniform large beam diameter illumination of the treatment area, thus eliminating the guesswork in determining the dose.